This Month’s Featured Article
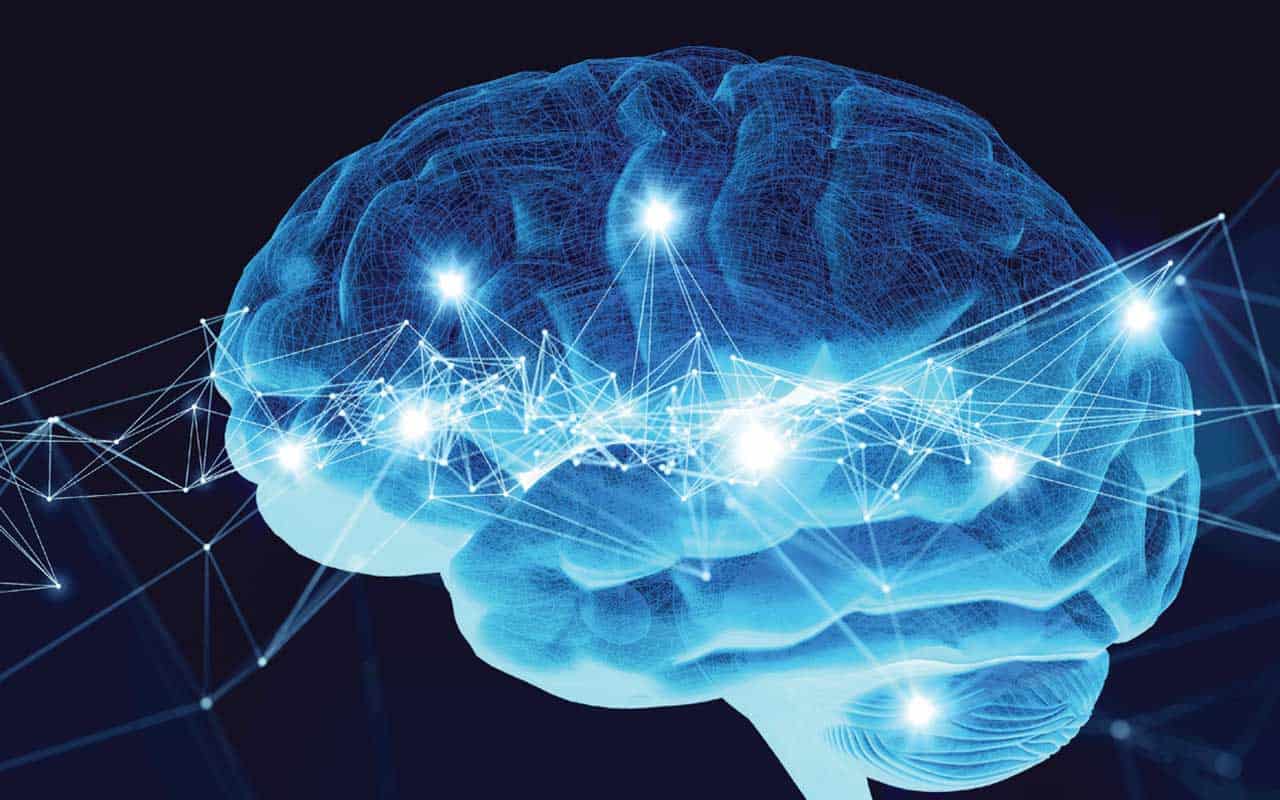
A Grey Matter
Here is something I know: there is a statue of Roger Sherman located in the rotunda of the National Capitol in Washington, DC. There is a likeness of a notable person from every state located there, and when one takes a tour of the Capitol building, the tour guides make a point of trying to find the one from your state. I don’t think I will ever forget this fact.
Because, while I was on that tour, I heard a gunshot report around the rotunda. Instinctively, I grabbed my daughter and our grandfatherly tour guide and pulled them toward the nearest exit as tourists scrambled for cover. We ducked behind the nearest doorway and glanced back into the rotunda to assess our next move.
You probably never heard about this in the news because, as it turns out, the gunshot was just the sound of a tire exploding on a baby stroller. But in that particular location, having gone through several rounds of security to reach the rotunda, I was primed to think it might have been a gunshot, and I responded accordingly – almost instinctively – without a second’s thought.
And therein lies the wonder. How is it that I knew to respond to that stimulus in that particular way. And why is that memory so vivid and unforgettable? In the past, we might have chalked it up to simple instinct, but instinct alone doesn’t explain my actions.
From event to memory
Let’s picture it again in more detail: when I heard the sound, the stimulus entered my ear, sending information to the center of my brain – the hypothalamus – which, in turn, sent a signal to the adrenal glands, located just above the kidneys. Almost instantly, adrenaline coursed through my body via the nervous system, dilating blood vessels and increasing oxygen to the lungs, which in turn primed my body for a physical response. Biologically speaking, it all sounds very orderly.
How I developed a memory of that event, however, is far less orderly. The vast majority of experiences vanish from our brains, yet certain events such as my day at the Capitol or, say, the day my family moved away from our childhood home, are indelible. Even more puzzling is how other, less emotionally-charged thoughts retain their spots in our mind. Why is it that I can remember the phone number of my best middle school friend, but I can’t keep straight my Netflix password?
Over the past twenty years, researchers, aided with innovative technology and more computing power than the world has ever known, have begun to understand how our brains work with greater depth and precision. Tools such as functional magnetic resonance imaging, or fMRI, have helped scientists to actually see learning as it happens, and to assess the way that various stimuli engage the parts of the brain that are responsible for our cognitive processes (not to mention our non-cognitive processes … more on that later). The result is a new understanding of how our brains operate that is revolutionizing classrooms and learning.
The brain’s functions: the tale of Phineas Gage
Our understanding of the brain’s functioning is a relatively recent phenomenon. In 1848, a railroad foreman named Phineas Gage was preparing a hole for a blast charge on the Rutland and Burlington Railroad line when an inadvertent explosion fired a tamping rod directly upward through his brain and skull, exiting out the other side. Miraculously, Gage survived the blast and could even talk with his attending physician as he tended to the wounds.
As Gage convalesced, however, friends and family began to notice changes in his personality. Although his memory seemed unaffected by the accident, aspects of his personality had changed dramatically. Once affable and congenial, Gage was “at times pertinaciously obstinate, yet capricious and vacillating,” according to his doctor. The particular path taken by the tamping rod seemed to affect some functions more than others, leading to the theory of “localization” in nineteenth-century medicine, which held that certain parts of the brain were responsible for certain operations.
Most of us today are generally familiar with the fact that certain regions of the brain control particular functions, and even with the notion that there are “left-brained” and “right-brained” people, with the former excelling at analytical, logical activities, and the latter in touch with their more emotional and artistic selves. The reality, however, is that there are numerous parts of our brain that all need to operate cooperatively in order for learning to happen, and
it is both this fragmentation and cooperation that make the brain fascinating.
We owe a lot of our understanding of the brain to gross experimentation on epileptics and other afflicted individuals who paid the price for their ailments with lobotomies and experiments that may not have cured the patient but resulted in incremental advances in our understanding of cerebral activity. In one such case, a doctor removed a portion of a patient’s brain and discovered that while he had cured the patient’s seizures, the patient lost all ability to form new memories. Even five minutes was too long for the patient to retain information about a conversation, even as it was happening. Conclusion? I guess we really needed that little piece of grey matter. Over time, doctors and scientists began to identify the specific parts of the brain that governed its various processes, but only with great cost to patients and painstaking, methodical work on the part of the scientific community.
The advent of MRI
With the invention of the MRI in the late 1970s and eventually fMRI, however, scientists acquired the ability to see cognitive activity in real time. Here’s how it works: when the brain works, it demands increased blood flow to the areas of the brain that are doing the work. Blood contains iron, which generates slight variations in the magnetic properties of the brain as blood flow increases. Those magnetic fluctuations can be captured by highly sensitive imaging devices, and voilà: magnetic resonance imaging machines. The “f” in fMRI means that the image is recorded while the brain is functioning. An operator asks a patient in an MRI machine to perform a cerebral function of some sort while he records what is happening inside the brain. In certain machines, this could even include audio/visual stimulation to prompt a cognitive process while the machine scans the brain every second or two to create an image of it as it works. Show the subject a scary movie, and you can watch certain areas of the brain light up in response.
The triune brain
Thanks to these advances, we now know that at the most basic level, human beings have triune – three-part – brains. The area around our brain stem at the top of our spinal column is the most primitive part of our brain and probably the first to develop, evolutionarily-speaking. When I was in the Capitol that day and heard what I thought were gunshots, this part of my brain, which includes the hypothalamus, processed the sensory data and bypassed cognition to dictate my next move. “Fight-or-flight”” responses and instinct emanate from this part of the brain.
The area surrounding the hypothalamus contains several distinct areas that comprise the limbic system, which is responsible for emotions and learning. Most important among these are the hippocampi, two small, curved organs that connect emotions to memories and learning. As information enters the brain, the hippocampus acts like a mail room, sorting messages where it thinks they belong according to previous experience. It is constantly comparing and contrasting new information, making analogies to other things we have done, know or – most importantly – felt. This interplay between emotion and learning is one of the biggest takeaways from fMRI-informed research and one that is spurring tectonic shifts in modern classrooms.
Any kindergarten teacher has seen firsthand how emotions impact learning. Young students are forever calling their teachers “Mommy” by accident because they feel cared for by a loving female adult who nurtures them in much the same way as their mother. The limbic system compares this caring adult female with the relatively few others it has known (i.e., their mother), occasionally resulting in a Freudian Slip. Eventually, children meet more and more female teachers, and the brain begins to process them appropriately by frequently and repeatedly contrasting them with their mother. Over time, their neural pathways strengthen to facilitate the processing of other females with greater accuracy and speed.
These acts of comparison and contrast gradually become noetic (conscious and intentional) and migrate to the neocortex, the most evolved area of the brain that blankets the other regions and takes responsibility for higher-order thinking. There is still much that we need to learn about this part of the brain, but we do know that it is responsible for activities such as sensory perception and language processing, and that decision-making takes place in the neocortex with information provided from the other regions. It is the last area to develop in humans, and when teenagers make poor decisions about … well, anything and everything, the neocortex is often where the breakdown occurs. It just isn’t fully functioning yet.
Neural pathways and brain plasticity
Teens are notoriously accident-prone drivers largely because they are inexperienced. In neurological terms, that means they have not yet developed the neural pathways that support better car-handling. When a teen hits a patch of ice for the first time, the brain must instantly process the input and make a split-second decision based on previous experience and learning. If, as in Canada, that teen has been taught to steer into the turn by practicing on a frozen lake, there is precedent for a better decision. And as that teen becomes an adult, more and more icy escapes begin to strengthen the neural pathway. It is similar to a twig that grows into a branch on a tree – it will eventually reach a point where it is nearly unbreakable.
But not entirely. Over time, the brain is capable of physical changes as a result of new learning and experiences. Throughout the brain, cells called neurons perform the function of sending information to other neurons through networks of axons and dendrites. Axons and dendrites are the pinnate features of neurons that communicate to one another through chemical and electrical signals called synapses. With each use, axons become stronger through myelination, a process that insulates the axon with a fatty substance that promotes faster exchange of information and reduces interference. Thus, the brain grows.
This plasticity, or ability to change, grow, and evolve is most dynamic in young people, but it characterizes our brain throughout life. We are always capable of developing new neural connections, but as we get older, it gets harder and harder to do that because our existing pathways are so well-established. We may not be able to prune that branch, but if it falls into disuse, we may strengthen other neural pathways instead. For instance, those of us who are “digital immigrants” have been able to adapt to this highly digital world, though we may not be as facile with technology as the “digital natives” of Generation X and later.
Implications for teaching and learning
fMRI technology has only been available to scientists since the early 1990s, and the field of affective neuroscience is even younger, so our understanding of the implications of this research for teaching and learning are still nascent. Educators are following the bread crumbs. However, to innovative classroom practices that capitalize on some of the new information in both logical and in somewhat unexpected ways.
Motivation is a classic attribute of the successful student, and as we learn more about how our brains produce a feeling of motivation, we are better able to tap into this essential ingredient in the learning process. Neurotransmitters such as dopamine fuel our brain’s interest in learning new things by responding to new experiences and the intrinsic rewards associated with mastering new concepts. Dopamine specifically causes us to want something, although current research suggests that its power is more in anticipation than reward. Thus, teachers who introduce novel topics (or old topics in novel ways) or who integrate innovative practices in their classrooms tap into our natural dopamine production to motivate students.
Dopamine production occurs when learning engages our “seeking” functions in several different senses. In addition to seeking new learning and information, our brains are essentially wired to seek answers. Projects that pose relevant, engaging questions or challenge students to design solutions to challenging authentic social problems tap this part of our nature. In addition, social interaction has been found to stimulate and enhance our drive to seek, so collaboration is another important feature of well-designed lessons.
Norepinephrine is another neurotransmitter, but instead of stimulating brain activity, it works to regulate certain bodily functions such as blood pressure and heart rate in response to stimuli. I benefited from norepinephrine during my experience in the rotunda when it primed my physical systems for “fight-or-flight” thinking and my neurological systems to access working and long term memory. In an instant, I was able to call up memories of 9/11 and hostage movies, and I used them to select my next move.
Countering the effects of chronic stress
Chronic exposure to stress, however, overloads neurological pathways related to physical movement and emotion, and it can limit the growth of more cognitive functions. Students who experience trauma in early childhood often lack a fully-developed prefrontal cortex because so much of their brain activity takes place closer to the hypothalamus. As a result, psychologists have developed the Adverse Childhood Experience Study (ACES) as a way of easily identifying students who require intervention to counteract the effects of chronic stress.
If there is a single ground-breaking conclusion to be drawn from the research however, it is the intimate connection between emotion and learning. Indeed, a recent publication by educational heavyweights Douglas Fisher and Nancy Frey was entitled All Learning Is Social and Emotional, just to underscore the role that these factors play in academic success. When we account for a student’s emotional preparedness for learning, teach them self-regulation skills, and engage their social and emotional aspects, we anchor learning to emotions in the way the hypothalamus does. We prepare them for greater success in life by teaching them not only the academic information they need, but the emotional and social skills that make that information useful.
Several programs are designed to do just that. One program from the Yale Center for Emotional Intelligence helps students to name their emotions through daily practice with the Mood Meter, a colorful square with quadrants for plotting one’s emotions throughout the day along two poles: high energy/low energy and pleasant/unpleasant. The program is designed to help students identify, name, and perceive their emotions in order to make them more aware of them as they happen as well as the antecedents and conditions that affect them.
Designing better instruction: Next Generation Science
Additionally, educators are transforming traditional classroom structures to align them with current research about the brain. Science classrooms, in particular, have undergone dramatic transformations as teachers adopt Next Generation Science Practices. Most of us experienced very teacher-centered science courses: an instructor demonstrated an experiment from the front of the room, and we were tasked with replicating it with our lab partner, who may or may not have been paying attention.
Today, science instruction alternates among “anchor phenomenon,” discussion periods, and opportunities for students to theorize, test their thinking, and reason. The anchor phenomenon is novel for students and takes advantage of the dopamine rush that ignites our “seeking” system. Why is it that an inflated balloon explodes when you peel an orange above it? Why will termites follow ink lines on a piece of paper? Is it true that a tanker truck can implode? How does that happen? Students work together to investigate these phenomena. Engaging the power of collaboration and social interaction. They work alongside a skillful teacher who is trained extensively in how to track their conversation to identify misconceptions before they become anchored in our neural pathways, requiring even more work to correct. Lastly, they create their own experiments to test their hypotheses, which taps into our brain’s desire to solve problems and experience something new and different.
Dr. Jaak Panskepp, a researcher at Washington State University, has pioneered the field of affective neuroscience to study the interconnection of brain function and emotion. Much of the research I presented here can be traced to his work. According to him, the desire to seek, which is connected to the anticipatory rush of dopamine to the neocortex, is the key to unlocking motivation and driving learning. “[T]his system, so important for generating feelings of ‘enthusiasm’ as opposed to rewarding ‘pleasure,’ needs to be on the radar for educators. If this system can be captivated by teachers, they have done half their job.” The rest of it is up to the brain.
By Ian Strever | info@mainstreetmag.com